Niels Bohr (1885 – 1962) was a Danish physicist who significantly improved our understanding of the structure of the atom. His improved model of the atom solved the problems associated with classical physics and was based on the newer quantum mechanic physics.
Early Life and Education
Niels Bohr was born on October 7th, 1885 in Copenhagen, the capital of Denmark. He was born into an intellectual and supportive family, and his upbringing consisted of a rich educational environment. His father, Christian Bohr, was a professor of physiology at the University of Copenhagen. His mother, Ellen Bohr, came from a prominent Jewish-Danish banking family with influence in parliamentary circles. The combination of his fathers scientific work and his mothers cultural pedigree together created a home environment that nurtured curiosity and scholarly pursuit. It is no surprise that young Niels Bohr grew up strong in mathematics and was naturally attracted to science at a young age.
In 1903 he enrolled as an undergraduate at the University of Copenhagen, where he received his master’s and doctorate in physics by 1911. That same year he traveled to England to work in the Cavendish Laboratory where he met J.J. Thomson. The two scientists didn’t work well together at first, but Bohr soon met another scientist at a different laboratory whom he worked better with. In March 1912 Bohr traveled to Manchester to work with Ernest Rutherford, who had recently won a Nobel Prize in Chemistry for his work on radioactivity.
Scientific Career
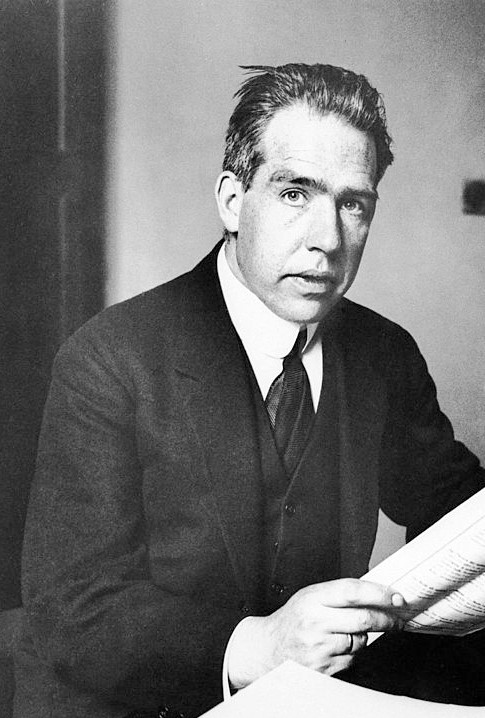
Bohr returned to Denmark in 1912 after having secured a teaching position at the University of Copenhagen. He brought with him new ideas about the structure of the atom. It was becoming clear that Rutherford’s model of the atom, based on classical physics, was unstable. According to classical physics, the electrons moving around the nucleus of an atom in orbit would emit electromagnetic radiation, causing the electron to lose energy and eventually spiral into the nucleus. The form of electromagnetic radiation being emitted came to be called photons, with each photon having its own precise wavelength and amount of energy. Quantum physics states that objects emit photons in discrete packets rather than in continuous streams. Using quantum physics, Bohr proposed that electrons are confined to fixed orbits, each with their own distinct energy level. They only suddenly jump to lower or higher orbits as a precise amount of energy is emitted or absorbed in the atom. For example to move an electron to a lower energy level it emits a photon of the precise amount of energy that is the difference between the two orbits. Using Plank’s constant, the frequency of photons, and some information about the electrons mass and charge, Bohr was able to obtain an accurate mathematical formula for the hydrogen atom. In 1922, Niels Bohr was awarded Nobel Prize in Physics for this work.
Bohr continued to work on quantum physics for the remainder of his life. He founded the Niels Bohr Institute at the University of Copenhagen. Later in life he was a part of the Manhattan Project during the Second World War, working with many other great physicists. He died in Copenhagen at the age of 77.